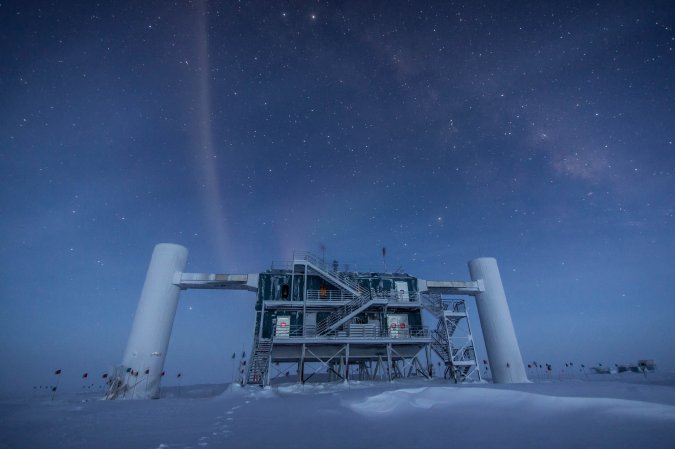
The neutrino has been called “the ghost particle,” due to its otherworldly ability to pass through solid objects, including you and me. Researchers in Antarctica are trying to catch them, hoping they might reveal the most energetic areas of the universe.
Neutrinos: you can’t feel them, you can’t see them, but they’re everywhere. They’re constantly zipping around and through us: in a single second, about a hundred billion neutrinos pass through the square centimeter of your thumbnail. They come from far — the very edges of the universe — and relatively near — the sun is a neutrino factory — and the overwhelming majority are nearly impossible to detect.
Like a proton, neutron or electron, a neutrino is a subatomic particle — but a very mysterious one. What we do know about it is strange: it is very, very, very light (nearly massless), possesses no electric charge, comes in three types or “flavors” (electron, muon and tau), can switch flavor mid-flight, and rarely interacts with any other particles. And these strange particles just might hold hope for new discoveries about our universe. “They’re fantastic cosmic messengers,” says Dr. Jenni Adams, associate professor of physics at the University of Canterbury (TEDxChristchurch talk: A mountain-sized telescope, under a mile of ice). “They can travel from the edges of the universe and from the most dense objects in the universe.” She details the race to understand the neutrino — and the giant physics experiment currently taking place deep in Antarctica.
The neutrino is a relatively new discovery. It was first identified in 1956 by Clyde L. Cowan and Frederick Reines, who found the neutrino by studying beta decay, a radioactive process that unstable atoms can use to become more stable, at the Savannah River nuclear plant in South Carolina. (They were awarded a Nobel Prize in 1995 for their work.) Ever since that discovery, physicists have been trying to make sense of them.
Intriguingly, for particles that exist in abundance everywhere, they’re incredibly difficult to detect. One of the reasons they’re so cool — the fact that they can pass through solid objects — also means you need to have a lot of patience before you can see one. “In some ways, detecting a neutrino is like winning the lottery,” says James Madsen, chair of the physics department at University of Wisconsin-River Falls (TEDxUWRiverFalls talk: Neutrino Astronomy: Can You See What I See?). Physicists increase their chances of seeing a neutrino interaction by building giant “traps” for neutrinos — machines that take up a lot of space and have a lot of neutrino targets (a.k.a. atoms). “What you can do is ask, ‘If I have so much material, what are the chances [a neutrino will hit it]?’” Madsen says. “In the same way that I can calculate the odds of winning the lottery — say it’s one in a million — and guarantee I will win if I buy all million tickets, the more material you have, the better odds you have of a neutrino interacting.”
The best neutrino detectors are clear, big and deep underground. You can find them beneath the Earth’s surface in gold mines, nickel mines, salt mines — and also Antarctica, where the neutrino detector that Adams and Madsen work on is located. That giant neutrino detector is called the IceCube; it occupies one cubic kilometer of South Pole ice (fun fact: if that ice were thawed, it could fill one million swimming pools). “When a neutrino does interact with an atom in the ice shelf, it produces charged particles, and those charged particles produce light,” says Adams. Antarctic ice is the perfect neutrino target, she says, because it is extremely clear. “Most ice contains air bubbles that would distort IceCube’s measurements,” the team says, “but the South Pole ice sheet is very thick and tightly compressed” so it’s ideal for detecting light.
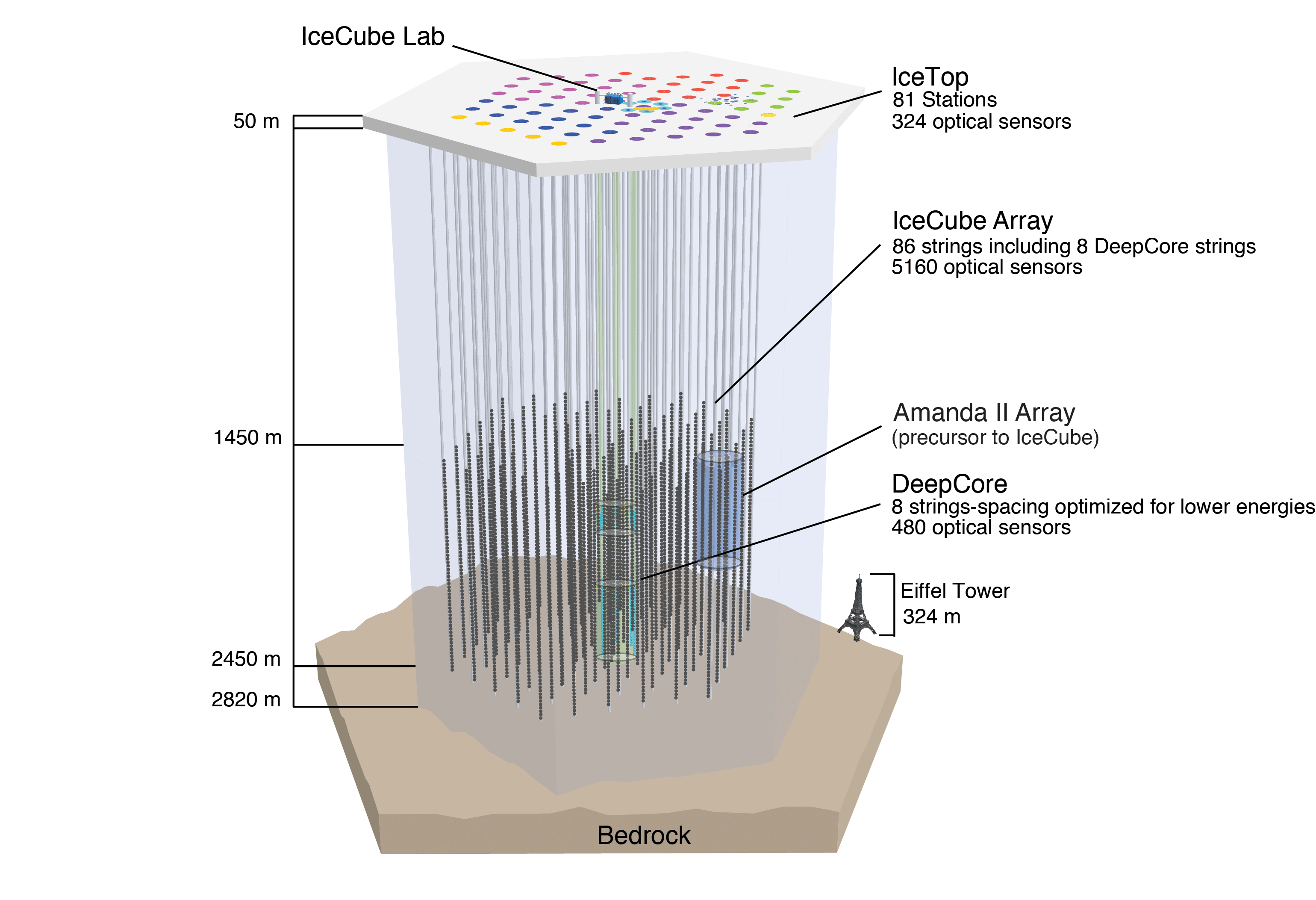
The IceCube Neutrino Observatory instruments a volume of roughly one cubic kilometer of clear Antarctic ice with 5,160 digital optical modules (DOMs) at depths between 1450 and 2450 meters. The observatory includes a densely instrumented subdetector, DeepCore, and a surface air shower array, IceTop. Image courtesy of IceCube.
Designing and building the IceCube was itself a massive undertaking. “We drilled 86 holes in the ice, and the distance between one hole to another is 125 meters (410 feet), or more than a football field apart,” Madsen says. “The depth of a hole is the equivalent of eight Eiffel Towers, and it took about 48 hours to drill each hole.” Construction took seven years — in part because workers could only be in Antarctica three months a year due to the punishing climate — and the facility began taking data in 2010. The machine relies on 5,160 extremely sensitive light detectors, round sensors the size of basketballs, to track the flashes of light produced by neutrino interactions in the ice shelf.
Adams and Madsen and the 298 other researchers at IceCube are trying to see if neutrinos can lead them to the unknown source of ultrahigh-energy cosmic rays. Cosmic rays are common occurrences — they consist of collections of particles (mostly protons) that have traveled from space and collide with the atoms in the Earth’s upper atmosphere. These interactions create a flurry of new particles, most of which are absorbed by the atmosphere before they reach our planet’s surface. Out of all the cosmic ray particles, neutrinos are the most likely to reach the Earth because of their unusual ability to avoid interaction. IceCube is designed to track any neutrinos that interact with anything in their cubic kilometer of Antarctica ice. Researchers have been crossing their fingers to find neutrino evidence of ultra-high-energy cosmic rays — super, super powerful (and super rare) showers of particles sent to Earth at energies millions of times more powerful than Switzerland’s Large Hadron Collider.
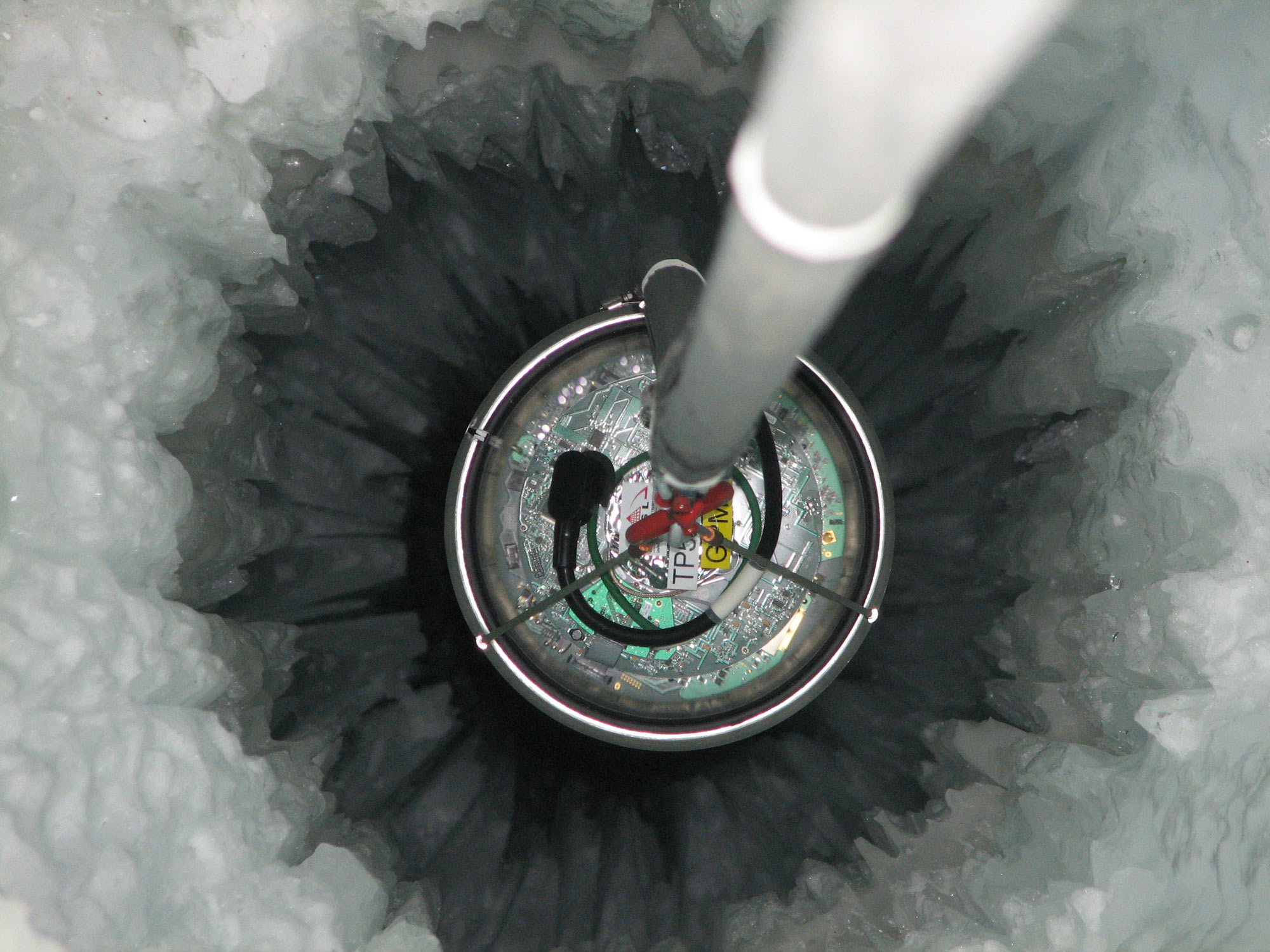
Could IceCube-detected neutrinos point us to the most energetic areas of the universe? Well, that’s the hope. Physicists think that humans may never be able to generate the energy of whatever accelerator is behind these ultra-high-energy cosmic rays. Particle physicist Ralph Engel of Germany’s Karlsruhe Institute of Technology estimates that with current technology, we’d need to build a particle accelerator the size of Mercury’s orbit (more than 223 million miles) to reach the energy of particles in these ultra-high-energy rays. So where are they coming from? Supermassive black holes? The decay of cosmic strings? Quasars? We don’t know. Adams says neutrinos are essential to this search: because they don’t interact with the electromagnetic force and therefore their paths aren’t bent by magnetic fields, they might point to where these rays are coming from — essentially act as a treasure map to this unknown cosmic powerhouse.
In Antarctica, the detector has found some extremely energetic neutrinos (hooray!) — but not the answers they were hoping for (yet). “There was an idea that once we found these neutrinos, we’d solve this mystery,” Adams says. But it hasn’t been that easy. “Now we’ve detected many neutrinos — in the order of 100 that we can say for sure come from high-energy astrophysical processes [rather than from the sun] — but when we look at where they’re coming from, they don’t seem to be lining up with any known objects,” like supermassive black holes, supernova remnants, blazars, or active galactic nuclei, she says. Which means there’s much more searching to do. “There are actually not many neutrinos from high-energy astrophysical objects, and there’s probably more than one type of object that these neutrinos are coming from so that makes it difficult to find a correlation with objects — because you have to get enough neutrinos from a certain type of object,” she says.
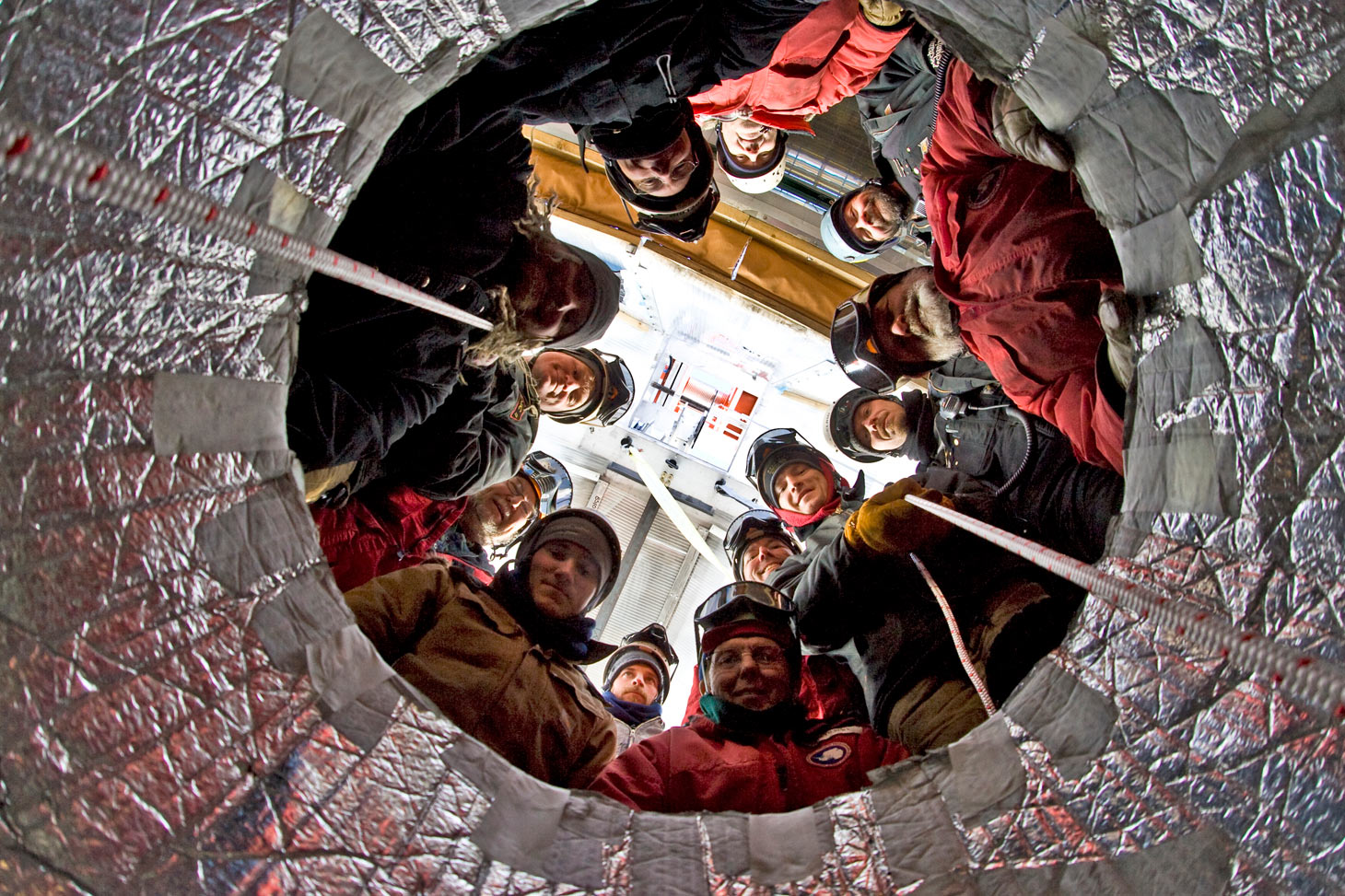
Still, significant discoveries have been made in the deep South. Researchers have “caught” the highest-energy neutrinos ever detected. They’re working with LIGO — the lab that confirmed the existence of gravitational waves — to search for neutrino emissions from colliding black holes. They’ve inspired NASA to go on the hunt for the birthplace of one of their most famous neutrinos — nicknamed Big Bird — and the NASA team thinks it might have a prime suspect: an unusually bright galaxy called PKS B1424-418. “We know something’s out there producing these tremendously high-energy particles,” Madsen says, “but still we don’t know where, or how, they are getting these high energies. It’s almost like someone’s stealing all your money and all you know is that your money is gone — you don’t know who is doing it, how they are doing it or where they are.”
IceCube researchers think we can find out where these cosmic rays come from — and that we can learn more about these elusive particles along the way. Here are just a few reasons to keep an eye on neutrinos: Scientists believe understanding them could help us figure out why matter won out over antimatter (neutrinos may be their own antiparticle), what exactly happens inside a star as it explodes (neutrinos are amazing escape artists that can get out of places from which nothing else can escape, like supernovas and the core of our sun), and what is going on outside of the Milky Way galaxy (neutrinos may be our guide to intergalactic energy powerhouses). “Something is managing to accelerate these particles to much higher energies than we can on Earth,” Adams says, “and at the moment we don’t even know where in the universe this is happening or what kind of object is doing that. IceCube is a way to understand the highest-energy processes in the universe, and neutrinos are key to that.”