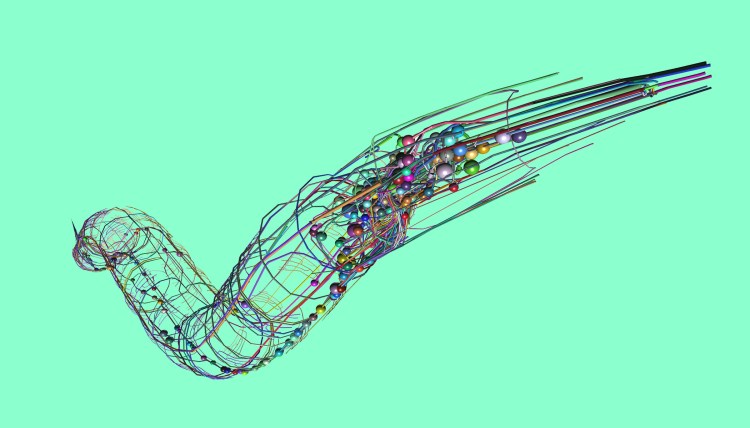
An open-source project is striving to create the world’s first fully digital organism. Here’s a peek inside the tricky process that could bring us to a better understanding of life itself.
A genius scientist creates an artificial being, it somehow gains free will and consciousness — and then goes rogue. That’s been the basic plotline of stories and pop culture from Frankenstein to Westworld. But outside of novels, movies, TV and people’s vivid imaginations, is it truly possible for humans to create a an organism that “lives unscripted”?
That’s what Stephen Larson, a Boston-based neuroscientist, entrepreneur and CEO of Metacell, a neuroscience software company, and an international team of over 100 scientists, designers and programmers set out to do when they formed the Open Worm Project (TEDxBangalore Talk: A worm is the best way to unlock the secrets of the brain). Their mission was to create the world’s first digital organism that uses the principles of life to exist on a computer — and to do it as a public, open-sourced project (work is coordinated on Google hangouts, and anyone can eavesdrop on their meetings on YouTube).
As their test case, the team chose the Caenorhabditis elegans worm, the world’s most understood multicellular organism. This one-millimeter-long roundworm is a favorite of scientists because it’s the simplest animal with a brain to study. Its entire body consists of 959 cells, of which 302 are neurons that form approximately 10,000 nerve connections. (By contrast, the human brain contains around 86 billion neurons and 100 trillion synapses.) Yet even with a small neural network, the worm can feed, mate and evade predators and toxins.
C elegans was also the first multicellular organism to have its genome sequenced in 1998, giving biologists a full understanding of how it develops from embryo to adulthood. A database called Wormbase is a constantly updated clearinghouse of genetic and biological studies of the worm’s molecular functions. And, last but not least, the tiny worm is the only organism for which a “connectome” — a 3D map that shows how each of the nerve cells is connected to each other — has been made.
The project has called on a convergence of neuroscience, computational technology, and a relatively new field called virtual cell biology. Over seven years, the team members have converted the behavior of each cell and how it functions into an equivalent mathematical system of differential equations. Gepetto — a web-based, multi-scale platform that they designed and built for this Project — then integrates the multiple simulation algorithms together into a single biological model.
Of course, their efforts to construct a virtual organism have faced one enormous obstacle. Nobody — on the Open Worm Project or outside it — has figured out how to build living cells starting from the molecules up. So the team decided fairly early on that they had to opt for behavioral rather than biological realism and focused on recreating the activity of individual cells to render overall behaviors. “Think of it like a Pixar character, except not scripted,” says Larson. “We want the cells to be the animator.”
But even creating behaviorally realistic cells that can be “the animator” is complicated. For example, consider the knowledge required to create a replica of each of the worm’s neurons and its corresponding function. Scientists believe they know what neuron must be triggered to cause a behavior, but they don’t know exactly how a neuron conveys information in the brain because activity takes place along breathtakingly complex networks. “We can observe biology by isolating,” explains Larson. “We know a neuron will send a chemical signal across a synapse and how one neuron affects one muscle. But how neurons come together, all doing their parts to create the whole behavior? We can’t see the forest for the trees.”
Right now the Open Worm’s neurons are able to signal but the activity is occurring at a grossly simplified level. Larson describes it as having a “very low resolution” at this point. Yet the researchers continue to add more and more data to their model, and as the simulation gets more and more mathematically intricate, more detailed algorithms are being created to simulate cell behavior. But constructing this virtual organism, it turns out, is less like building a house by nailing and bolting together pieces and more like painting a picture of a house, making it visible to the eye and increasingly complex over time.
In 2015, a breakthrough happened: the team uploaded their worm’s brain into a LEGO-constructed robot — and the robot moved. Using an existing physical structure allowed for helpful substitutions. The worm’s nose neurons were replaced by a sonar sensor; the motor neurons on both sides of the worm by actual motors. Still, the software that the team created and which was modeled on the neurons of the worm’s nervous system, led to their robot hitting a wall, backing up and moving the other way. This was not a programmed act — it was all behavior derived from its connectome. Of course, making this activity happen inside the virtual body of a worm is a huge next step. Right now the worm’s body is essentially in one place and its mind in another. Remember — this creature is virtual, so the Open Worm team can work on various parts — the synapses, the musculature — separately. “There’s a team working on neurons, and a team working on the body,” says Larson. “Only recently have those two teams been running experiments together.”
Unsurprisingly, the project has attracted critics. They contend that the worm doesn’t offer any new understanding to neural behavior. With the inability to reproduce the basic molecular building blocks of life, they say, it will always be a simulation. What’s more, the C elegans connectome was based on the map of a sliced-up dead worm, not a live one, so critics also question the Open Worm’s neural fidelity.
One question that emerges from their criticisms is: How do you define whether something is alive? That’s a question that all scientists, not just on the ones working on Open Worm, are trying to answer. There are two primary camps: those who believe life requires the ability to reproduce or replicate itself, and those who believe it depends on the ability to survive. Larson belongs to the group that living systems must be able to self-sustain. So whether the Open Worm is correct on the molecular level doesn’t matter as much as whether it can feed itself or back away from harm. In fact, the latter is the next goal for the worm — the team wants to see its worm crawl forward, get tapped on the nose by a researcher, and crawl backwards. This would mean that after receiving a bonk on its nose, it would sense it, process it, and then create a neural signal to retreat, all on its own, which would be a first for simulated brain activity.
The Open Worm’s defenders explain their work does have value. It could help us understand how a worm works on a cellular level, information that applies to much more than C elegans. “The same alien technology that makes the worm work, the same moves and rules and events that happen inside it are the same kinds of events that happen in all of life across the planet,” explained Larson in a TEDxVienna Talk, Digital biology and open science. Even so-called failure could ultimately prove to be a kind of success. While the process of taking what we observe of neural circuitry and translating it into algorithms might not result in the construction of a perfect worm, it could lead to the design of powerful computer models that could analyze and synthesize information in unimaginable new ways. And in that way, discovering how several dozen neurons can make a worm crawl backwards could represent a major leap forwards for humans.