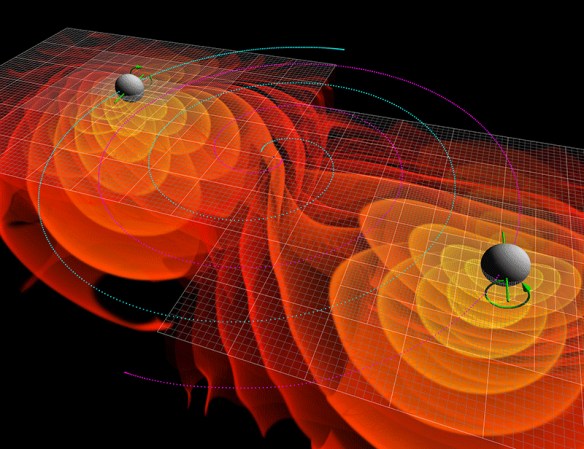
How do you build a real-world machine to test the most abstract of theories? Janna Levin talks with Rainer Weiss, one of the designers of LIGO, the four-km-long instrument that can detect the distant reverberations of two black holes crashing into one another.
Janna Levin is a theoretical physicist — she works with pen and paper to turn the elegant rules of the universe into theory (and also uses that pen and paper to write books about science; her latest, Black Hole Blues, was recently published by Alfred A. Knopf). Rainer Weiss, or Rai, as he’s known, is an experimental physicist — he thinks about how to find and measure something that may or may not exist outside of theory. Weiss was part of the group that designed and built LIGO, the detector that, in September 2015, for the first time “heard” the sound of two black holes crashing into one another, producing a gravitational wave that stretched spacetime and proving something that Einstein first floated as a theory almost 100 years ago. Earlier this week, the LIGO team announced that on December 26, 2015, they had detected another rippling, spacetime-stretching gravitational wave that erupted 1.4 billion years ago when two other black holes collided at half the speed of light.
In a sparkling conversation held at a Brooklyn art space, Pioneer Works, Levin and Weiss (with help from journalist John Hockenberry) talked about how they work, how LIGO works — and the moment when they realized Einstein had just been proven right. An edited version of the discussion follows.
The 50-year, billion-dollar bet
Janna Levin: The first detection made with LIGO was of a pair of black holes that collided 1.3 billion years ago. The success of the experiment was not only the detection of gravitational waves. That was the first time we detected a pair of black holes, because they’re dark and we can’t see them with telescopes. So it was really an astronomical discovery as well.
The detection happened in September 2015. In August 2015, Rai and I were talking and he said — this after the experiment cost a billion dollars, people had spent 50 years building it — he said, “If we don’t detect black holes, the thing is a failure.” I thought that was a really bold thing to say on the eve of starting the detection, because most people in the scientific community did not think we would detect black holes, at least not for a long time.
Rai Weiss: What was behind that statement — it is slightly facetious, of course — but you have to understand what black holes ultimately mean to people, and what they mean to the theory of gravity that Einstein put together. It’s the one thing that is so Einsteinian, we couldn’t have gotten it any other way. The whole idea of gravity curling up space, that is the epitome of what is going on in a black hole. I would’ve loved to have seen Einstein’s face if he were presented with the data that we actually discovered such a thing, because he himself probably didn’t believe in much of it.
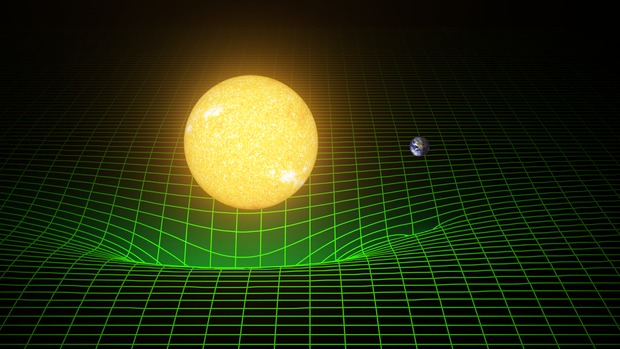
When black holes collide
JL: I’m a theoretical physicist. I only work pen-on-paper. I think that’s why I became so enamored of the physicality of the LIGO experiment. It’s one thing to say, “Oh, two black holes are going to collide,” and work it out on paper. It’s quite another thing to build giant L-shaped instruments that span four kilometers on two coasts.
RW: The equations don’t tell you much until you put actual numbers into them and you find out how much energy is involved in making a distortion of space. A gravitational wave is a very slight stretching in one dimension. If there’s a gravitational wave traveling towards you, you get a stretch in the dimension that’s perpendicular to the direction it’s moving. And then perpendicular to that first stretch, you have a compression along the other dimension. It compresses that way, and it stretches that way.
The problem is the energy that’s needed to make that very tiny little distortion in the fabric of spacetime. Space is much stiffer than you imagine; it’s stiffer than a gigantic piece of iron. That’s why it’s taken so damned long to detect gravitational waves: to deform space takes an enormous amount of energy, and there are only so many things that have enough.
JL: Those two black holes were orbiting each other probably for a few billion years, and they were creating gravitational waves the whole time, but the waves were weak because they were still far apart. It wasn’t until the black holes got within a couple of hundred kilometers of each other, in the final 200 milliseconds of their life together, when each one’s going at nearly the speed of light, and they collide to make a new black hole. It’s only that last fraction of this very long process that they’re able to detect at LIGO.
How to measure spacetime, stretching
RW: Here’s the fundamental problem when it comes to measuring the stretching of space: We’re looking for a motion that is 1,000th the size of a proton. It’s 10^-18, which is a decimal point, 17 zeros all in a line, and then a one. You can make the measurement by using a lot of light and an interferometer to look at things that are about 10^-18 meters of motion. You can do that. That’s the easy part. The hard part is how not to detect everything else around you — the people next to you, the leaves blowing on the trees in Louisiana, the winds blowing and howling up in Hanford, Washington. If you’re lucky, if the building is a good building, the amount of motion of the ground you’re dealing with is about a micron, it’s about 10^-6 meters. But you have to somehow get rid of that motion.
John Hockenberry: What were you doing when you thought of the first prototype of LIGO?
RW: I was teaching a course. I was trying to explain what Joseph Weber, the man who first did these gravity wave experiments, was doing, how he had devised a technique I couldn’t understand, and I couldn’t explain it to the class. So I sat and sat and sat, and thought: How can I make the most pristine, simple-minded explanation of how you could detect a gravitational wave? It was very straightforward — at least maybe to me it was: Let me launch a big mass off in space, and put a good clock on it, and over here, I’ll launch another one with another good clock. Then we’ll measure how far they are apart — we’ll send a light beam from one to the other, figure out when it hits, measure it and see what the clock says. Then let the gravitational wave come down, which stretches and compresses space, and see how that changes that time. That was the basis of the idea. I gave that as a problem to the students in the class, and they all were able to do it, and then I put it aside. But in 1971 or so, that’s when I took this idea seriously — I said, maybe this idea is okay. So then it became, how would you do it in a practical way?
JL: When I first went to see the LIGO facility, I was blown away. There’s a sort of corner lab, and it’s a cold room, a clean room, so you go in in bunny suits and laser goggles. And that holds the apex of the machine where a laser comes out and it gets split and sent down two arms. The arms punch right outside the lab in an L-shape, so they cut right out into the — in the case of Hanford, the desert, and in the case of Louisiana, the swamp. Those arms are four kilometers long in each direction, and they just go. There’s not much between the apex and the end stations. So you take a truck, you drive four kilometers down to the end station, and there’s another much, much smaller lab which houses a chamber for the mirrors.
As in Rai’s original thought experiment, one beam of laser light is split into two, and the two beams head off in different directions toward mirrors at the end of each arm. The two beams should take the same amount of time to go out and come back to the apex. But a ripple in spacetime actually changes the distance each laser beam has to travel — so they don’t perfectly meet up back at base. By monitoring the interference pattern where they meet up, we can record the stretching and compression of spacetime.
[youtube https://www.youtube.com/watch?v=tQ_teIUb3tE&w=560&h=315%5D
Why LIGO is in more than one place
RW: To do the experiment, you had to have two sites, at least, in this country — and there are people all over the world building these very big Ls now. There’s another one near Pisa in Italy, another one that’s being built inside of a big mountain in Japan, and recently a decision was made to build another one in India. By the time 2024 rolls around, if things go as planned, there will be at least four detectors of about equal sensitivity all over the world.
There are two reasons we built two of them to begin with. The first one was that nobody would believe something that we only saw in one detector. We certainly wouldn’t have. The same waveform had to be in both detectors, and it had to have some information about where it’s coming from. Here’s this thing moving at the velocity of light, and coming from the southern sky somewhere, and it hit Louisiana first, and then about seven milliseconds, seven thousandths of a second later, it hit Hanford.
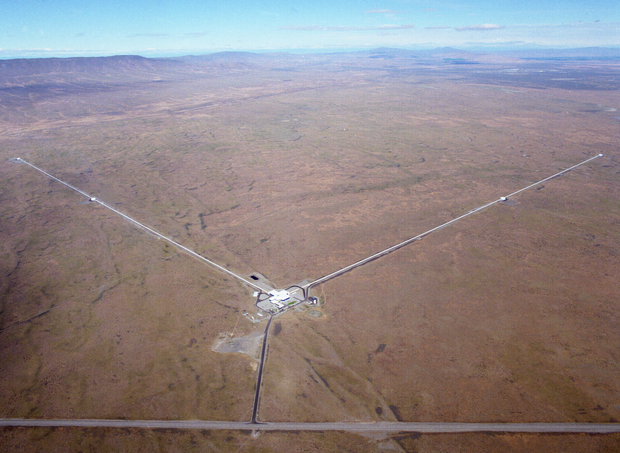
JL: This is really not a telescope, it is much more like a recording device, and so just as with all sounds, it’s much harder to identify where they come from. I mean, have you ever heard a ringing phone that you’re looking for but can’t see? Well, having two instruments is like having two ears, and the more instruments you have, the better you’re going to do.
Then we can start to understand which galaxy black holes lived in. And we can understand the age of the galaxy by looking at corresponding information from telescopes that can see that galaxy, even though they can’t see specifically the black holes.
[youtube https://www.youtube.com/watch?v=QyDcTbR-kEA&w=560&h=315%5D
What happened when LIGO heard that gravitational wave
RW: Many of us on the project were thinking if we ever saw a gravitational wave, it’d be an itsy bitsy little tiny thing, we’d never see it. This thing was so big that you didn’t have to do much to see it. To make it a little better, we filtered it, much like you do on your audio set when you don’t want to hear the bass rumble or you don’t want to hear the treble noise. You turn down the treble a little bit, you turn down the bass a little bit. That’s all that was done, and we saw these waveforms — you could see them by eye. That, alone, was the most stunning thing about all this — that the signal was that strong.
And what did we think when we saw a thing like that? First, it’s got to be a phony. Then, that looks really awful. You have to remember this was early in the run with a new detector. Everything wasn’t yet fully put together — it was working, but not all the software that we needed, all the bells and whistles were not yet together. But the instrument was good enough, and so some of us thought maybe somebody stuck that in there as a fake signal. We used to do that as a way of checking up on the people doing the data analysis, and as a way of testing the whole system.
Then people began to worry that maybe it was hackers who had got around a lot of the protections in place. And the most likely hacker would have been one of us, because we knew enough about the apparatus. But you would’ve had to make little features of the signal appear in the right places all over the apparatus, which means you would have had to know a tremendous amount about it. We took almost two weeks before we were really convinced that it wasn’t hackers.
JL: The day of the detection, the experiment wasn’t supposed to be in a science run yet. It was still under disruption, so people like Rai were there the weekend before looking for radio interference, which means they’re kind of banging on the machine — they’re interfering with the machine a lot. And students in the middle of the night were working on it, it was falling in and out of lock, meaning it wasn’t in observing mode. And then around four in the morning, Louisiana time, around two in Hanford, everyone gets tired, they all put their tools down, they keep the machine in observing mode, they go home, and within an hour, this thing that’s been traveling 1.3 billion years hits the Louisiana machine, goes those few milliseconds across the continent, and hits Washington. I just thought that was just a beautiful thing.
The collaboration kept this very, very quiet, they weren’t supposed to tell anybody, they’re supposed to keep it under lock and key. So I had a very touching moment when I received a note from David Reitze, the director of LIGO, and the subject line was, “Confidential communication from LIGO.” And my heart started pounding. I mean, I know what I’m about to read is going to change a lot. There’s going to be a moment before and after. And it was this beautiful note saying, “We have definitively detected the collision of two about -30 solar mass black holes from 1.3 billion years ago. It’s the first direct detection of gravitational waves, and the first detection of a binary black hole system.” And then he said, “Don’t tell anyone.” I’m trying to read it — my heart was pounding — and it was signed, “Dave, Rai, and Kip [Thorne].” That was just, for me, a really beautiful moment, scientifically, to be included in that. And then there were these months of just sharing it only within the collaboration, there was this closeness that I really think about, this sharing and excitement.
So … Has LIGO heard anything else?
JL: The machine is down at the moment, but when it’s back up again and tweaked up, the expectation is that there will be more. In the announcement, they did report another bump, which was probably a black hole pair, but it wasn’t loud enough to be an official detection. The hope is that there will be several detections per year, maybe even more.
What’s up with all the black holes?
JL: Black holes are not the engines of destruction that they’re portrayed to be. But technically, we are falling into a black hole. There is a black hole at the center of our galaxy, which is four million times the mass of the sun, we’re in orbit around it, which means we’re emitting a little bit of gravitational waves as we orbit. And some day, we’re going to fall into that black hole. But I think I’m much more worried about the election.